Imagination
Imagination is a straightforward form of virtual reality… what may not be so obvious is that our 'direct' experience of the world through our senses is virtual reality too.
-David Deutsch (1997, NP)
Can one dimension exist? I argue that there is no such thing as a single dimension or one-dimensional object outside of the mathematical concept. To test, we can try to make a mental picture.
Imagine two points in space.
Now imagine a line between those two points.
Does your line have any thickness?
In order to picture the line it must have some width or thickness; without it, the line would be invisible. Therefore, even in our minds, one dimension alone cannot be rendered. We seem to understand it as a component, one of three dimensions. This experiment reveals that a two-dimensional form can exist in our imagination. But can a two-dimensional form exist physically? Every flat surface has texture, has something supporting it, has some consistency. The closest thing to an actual two-dimensional form would seem to be a shadow—the absence of photons from a given light source inversely projected onto a surface. That shaped section of the surface is not directly absorbing, or reflecting light. We can measure its sides and define its area. Yet should we consider a shadow separate from the surface on which it appears? If there weren’t a three dimensional form for a shadow to appear on, would the shadow be two-dimensional?
For a moment let us consider how we experience the physical nature of light. We intercept light (think of your shadow on a sunny day); light can pass through us (remember seeing your hand glow red when put over a flashlight); a beam of light can pass through another beam of light; light is invisible until it strikes a surface,[1] substance or obstruction; light is reflected off of some surfaces and materials while it is filtered or absorbed by others.
Currently I sit facing north, it is 17:01 Central European Time. The room I am in is illuminated only by indirect sunlight. It is reflected off many nearby roofs, walls, windows, and trees, as well as a large section of the gaseous light blue envelope surrounding our planet—the atmosphere. Light enters the room by passing through glass windows and a very small amount of light emits from the computer screen on which these words form. Aside from this screen, the brightest surface in the room is a section of the wall to my left. Sunlight bouncing off the exterior walls and windows of the building just northeast of the one in which I sit is the predominant source of the glow on the wall. When I lift the computer monitor and carry it over to the wall, the light it casts multiplies on top of light reflected off the nearby building, occupying the same position. When I hit the wall with my hand, it does not pass through the wall. My hand cannot occupy the same position as the wall at the same time.
Quantum mechanics explains this observation with the Pauli exclusion principle. There are two types of particles: fermions and bosons. The Pauli exclusion principle states that two identical fermions cannot be in the same place simultaneously. Almost all familiar matter we encounter—a hand, skin, bones, a brick wall with plaster and paint over it—is composed of fermions, while light is composed of bosons. In other words, photons, the quanta of light, the smallest single units of light, are bosonic particles. Bosons of the same type can be found in the same place, the same quantum state. On the wall in my studio, I experience this principal, bosons on bosons, the possibility of photons building up in the same location. Yet when I run at the wall full speed and do a back flip into it, I do not melt into the wall. Fermions hit fermions. The electrons in my body cannot be in the same place as the electrons of the wall. In contemporary physics, electrons and photons are both considered elementary particles; they do not break down into smaller particles, yet clearly they are very different. A photon has no mass, and thus has the ability to travel as fast as it does: the speed of light.[2]
Knowing this, are we any closer to concluding whether or not there is physically such a thing as a two-dimensional form? Does light have dimension? For the moment I am satisfied with the shadow as the closest thing to two-dimensional, but it seems nearly impossible outside of mathematics to divide the three-dimensional world into its thirds, much less into elevenths.[3] Even if we could zoom in septillions of times smaller than the size of a photon, how might an extra dimension render itself? It seems easier to picture a world in which my hand goes right through the studio wall than an extra spatial dimension. Being invisibly small makes it easier to understand why we do not perceive the Kaluza-Klein dimension, but it does not make it any easier to visualize. Yet these dimensions exist. The fifth dimension exists in language, in equation, in theory and when the mathematical models of quantum mechanics don’t add up, its existence simply inspires physicists to imagine a sixth, seventh, eighth and so on.
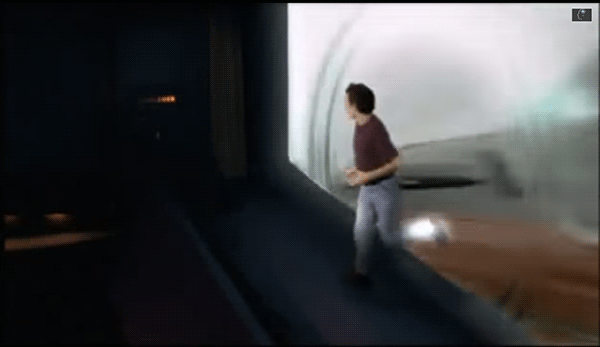
Video Still, The Elegant Universe, PBS, 2003
Theoretical physics conjures a non-visualizable world via logic at its limits: a model world blanketed by the gridded matrices of SU(3) holonomy, anti-symmetric tensors, Mexican hat potentials, Poisson brackets, Higgs fields, D-branes, heterotic gauge thresholds and one-loop superstring vacuum amplitudes. Physicist Lisa Randall writes in her book Warped Passages that, “as physics evolved in the twentieth century, it moved away from things that can be directly observed with the naked eye to things that can be ‘seen’ only through measurements coupled with a theoretical train of logic […] we know that dark matter and dark energy exist in the universe, not because we've detected them directly, but because they have noticeable effects on matter that surrounds them” (Randall, 2005, p.9). Much of theoretical physics will stay theoretical and many of its concepts and predictions will remain the invisible causes of phenomena unexplainable by classical physics. Yet some of these predictions will be and have been “discovered” or experimentally proven.
CERN, the European Organization for Nuclear Research, with its Large Hadron Collider (LHC), is the most complex, expensive and largest center for particle experimentation to date. On their website, one can find a brief text describing the graviton and the LHC’s role in potentially helping to prove the existence of extra dimensions: “If gravitons exist, it should be possible to create them at the LHC, but they would rapidly disappear into extra dimensions. Collisions in particle accelerators always create balanced events—just like fireworks—with particles flying out in all directions. A graviton might escape our detectors, leaving an empty zone that we notice as an imbalance in momentum and energy in the event. We would need to carefully study the properties of the missing object to work out whether it is a graviton escaping to another dimension or something else.” (Cern.ch, 2015). Take a virtual tour of CMS @ CERN.
Joseph Lykken, the theoretical physicist and collaborator at the CMS (Compact Muon Solenoid) detector of the LHC, in his lecture at the SETI Institute,[4] a non-profit research organization in California, explained the process of trying to discover the Higgs boson—a particle proposed in 1964 as part of the Higgs mechanism, a theory that, if proven, would solve many puzzles in quantum physics. Lykken says of the particles, “They are not sitting around, it’s not that you find them, you have to actually make them.” About every one in ten billion collisions of two high-energy protons in the LHC will produce a Higgs boson. Yet it only lasts or “lives” for about 100 yoctoseconds (0.000000000000000000000001 seconds). Then “it decays into other particles, so you don’t see the Higgs boson, you see the other particles.” It usually decays into two high-energy photons or into four leptons. These are referred to as “Higgs candidate events” because a Higgs boson particle is never directly detected: “When you see enough of these events, you convince yourself you are seeing Higgs bosons” (Lykken, 2014).
Both Lykken’s and CERN’s language are particularly interesting. Firstly, that the Higgs boson remains elusive—it is not the Higgs that is detected, but rather the photons or leptons it turns into. And the same goes for the soon-to-be graviton—it will be known by its absence. Secondly, the Higgs Boson is not “found” but rather “made” and the graviton “should be possible to create.” In a time when “indirectly observed” seems to equate to “experimentally proven,” and particle physicists fabricate the very particles they observe, science in this field can no longer lay claim to objectivity. “Truth is,” as Sci-Fi author and poet Ursula K. Le Guin puts it, “a matter of imagination” (1976, p.6).
[1] Its invisibility seems to be an almost paradoxical property considering the first definition of “light” in Merrium-Webster’s online dictionary: “something that makes vision possible” (2015). Source: http://www.merriam-webster.com/dictionary/light
[2] When Melvyn Bragg, from BBC 4’s In Our Time, asks physicist Frank Close “How do we know photons are there if they have no mass?” He answers, “Because they will transmit momentum and energy from one place to another.” Bragg responds, “So we know them by there effects?” Close confirms, “We know them by their effects” (BBC, 2015). Source: http://www.bbc.co.uk/programmes/b051vlpf
[3] Some versions of String Theory and M theory call for up to eleven dimensions (Kiritsis, 2007).
[4] SETI stands for Search for Extraterrestrial Intelligence. http://www.seti.org/.
Whatch the Lykken's lecture here: https://www.youtube.com/watch?v=FyHp90wAiXI
Table of Contents
Scale
Vanishing Exercise
Imagination
Procrustes
Through a Double Slit
No Dreams, No Logic
Erotics of Art and Science
Bibliography
Extra
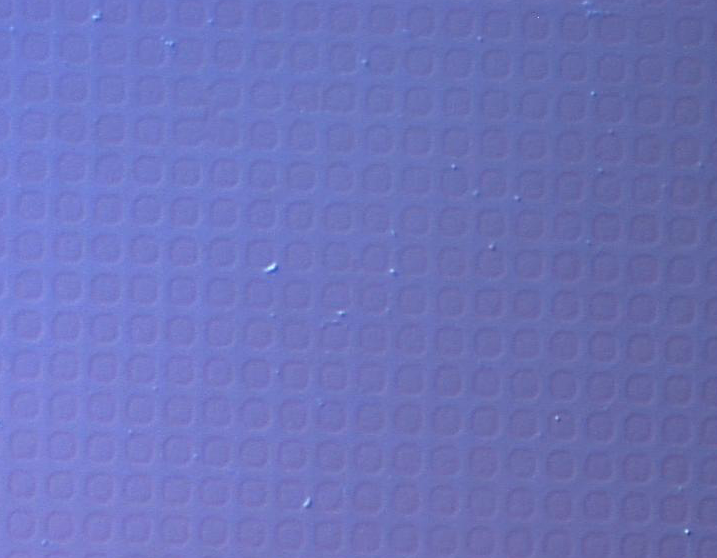
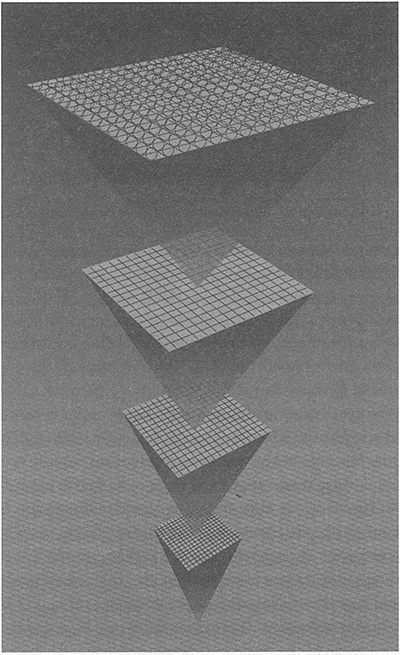
Figure 26. Each subsequent level represents a huge magnification of the spatial fabric displayed in the previous level. Our universe may have extra dimensions—as we see by the forth level of magnification—so long as they remain curled up into a space small enough to have as yet evaded direct detection.
- Brian Greene (Dawkins, 2008, p.340)
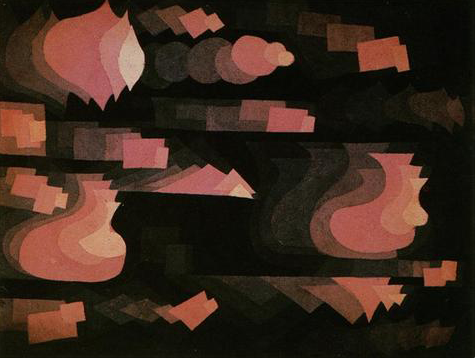
Paul Klee, Fuge in Rot, 1921
